Signal Integrity Requirements for Industrial IoT (IIoT) Cable Assembl...
Industrial IoT (IIoT) systems rely on seamless data transmission between sensors, controllers, and cloud platforms to enable real-time monitoring, predictive maintenance, and automation. At the heart of these systems are cable assemblies that must maintain signal integrity (SI) in harsh industrial environments. Compromised SI leads to data errors, communication failures, and costly downtime.
Why Signal Integrity Matters in IIoT
Signal integrity refers to the preservation of electrical signals as they travel through cables, ensuring accurate and timely data delivery. In IIoT applications, SI is threatened by:
Electromagnetic Interference (EMI): Generated by motors, relays, or nearby high-voltage equipment.
Crosstalk: Signal leakage between adjacent cables in dense installations.
Attenuation: Signal loss over long cable runs, especially in high-frequency applications.
Environmental Stressors: Temperature fluctuations, vibration, moisture, and chemicals.
Poor SI results in:
Packet loss in sensor data streams.
Delayed actuator responses in automated systems.
False alarms or missed critical events.
Key Signal Integrity Requirements for IIoT Cables
1. EMI/RFI Shielding
Shield Types:
Foil Shielding: Lightweight and cost-effective for low-frequency noise.
Braided Shielding: Higher coverage (70–95%) for high-frequency EMI suppression.
Combination Shields: Foil + braid for broadband protection (e.g., in PROFINET or EtherCAT cables).
Grounding: Proper shield termination to connectors or chassis to prevent ground loops.
2. Controlled Impedance
Impedance Matching: Cables must maintain consistent impedance (e.g., 100Ω for Ethernet, 50Ω for RF) to minimize reflections at connector interfaces.
Twinaxial Cables: Used in high-speed industrial protocols (e.g., CAN FD, SERCOS III) for balanced signal pairs.
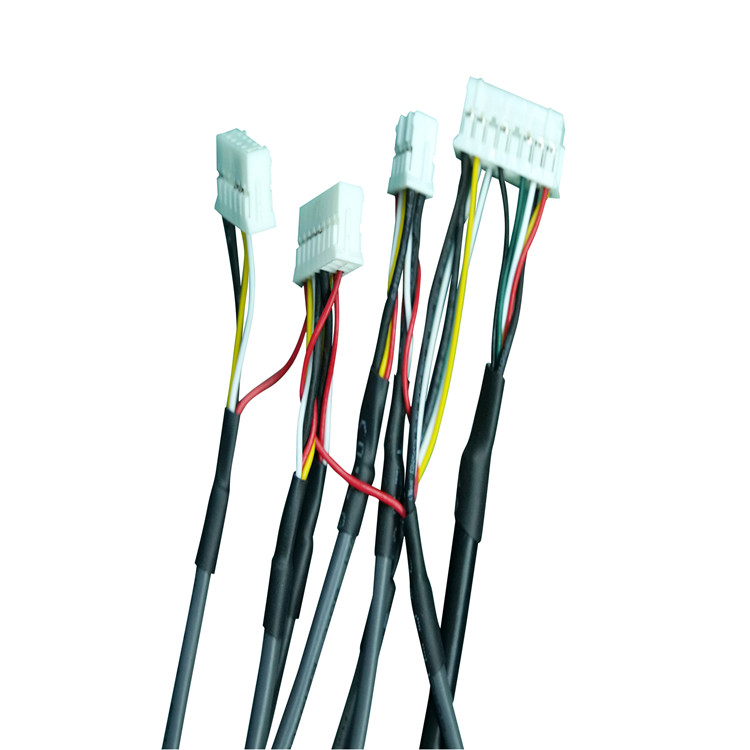
3. Low Capacitance and Skew
Capacitance: Minimize capacitance between conductors to preserve signal rise/fall times.
Skew Management: Ensure equal propagation delays in differential pairs to prevent timing mismatches.
4. High-Frequency Performance
Bandwidth: Cables must support frequencies up to 500 MHz for 5G-enabled IIoT or Gigabit Ethernet.
Material Selection: Foam PE or PTFE insulators reduce dielectric losses at high frequencies.
5. Durability in Harsh Environments
Temperature Range: -40°C to +105°C for outdoor or foundry applications.
Jacket Materials: PUR (abrasion-resistant), TPE (flexible), or FEP (chemical-resistant).
IP Ratings: IP67/IP68 seals for waterproof connectors in washdown areas.
Standards and Testing Protocols
IEC 61158: Fieldbus communication cables.
IEEE 802.3: Ethernet cable specifications.
TIA-1005: Industrial telecom infrastructure.
Testing Methods:
Time-Domain Reflectometry (TDR): Measures impedance discontinuities.
Vector Network Analysis (VNA): Evaluates insertion loss and return loss.
Eye Diagram Analysis: Tests signal quality in high-speed links.
Design Best Practices
Modular Connectors: Use M12/M8 connectors with D-coded (Ethernet) or A-coded (sensors) pins for secure mating.
Twisted Pair Geometry: Maintain precise twist ratios to cancel EMI in differential signals.
Ferrite Cores: Add clip-on ferrites near noise sources to suppress high-frequency interference.
Cable Routing: Separate power and data cables; use conduits in high-EMI zones.
Case Study: Smart Factory Ethernet Backbone
A German automotive plant upgraded its IIoT network with CAT6A SF/UTP cables featuring:
S/FTP (Screened/Foiled Twisted Pair) design for EMI immunity.
M12 X-coded connectors supporting 10 Gbps.
PUR jackets resistant to oil and coolant.
Results:
Signal attenuation reduced by 40% over 100-meter runs.
Latency dropped to <1 ms, enabling real-time robotic control.
Emerging Trends
Single-Pair Ethernet (SPE): Slimmer cables for IIoT edge devices (IEEE 802.3cg).
Fiber Optic Integration: Hybrid cables combining copper for power and fiber for data.
Predictive Maintenance Sensors: Cables with embedded strain or temperature sensors.